Temperature-Controlled Catheter Ablation for Paroxysmal Atrial Fibrillation: the QDOT-MICRO Workflow Sttudy
Tom De Potter1, Massimo Grimaldi2, Henrik Kjaerulf Jensen3, Josef Kautzner4, Petr Neuzil5, Johan Vijgen6, Andrea Natale712, Steen Buus Kristiansen3, Peter Lukac3, Petr Peichl4, Vivek Y. Reddy513
1OLV Hospital, Cardiology Department, Moorselbaan 164, 9300 Aalst, Belgium.2Ospedale Generale Regionale “F. Miulli” Strada Prov. 127, 70021 Acquaviva delle Fonti BA, Italy.3Department of Cardiology, Aarhus University Hospital, Palle Juul-Jensens Boulevard 99, DK-8200 Aarhus N, Denmark.4Institute for Clinical and Experimental Medicine, Vídeňská 1958/9, 140 21 Prague, Czech Republic.5Na Homolce Hospital, Roentgenova 2, 150 30 Prague 5, Czech Republic.6Jessa Ziekenhuis, Virga Jesse, Stadsomvaart 11, 3500 Hasselt, Belgium.7Texas Cardiac Arrhythmia Institute, St. David's Medical Center 3000 N. IH-35, Suite 720 Austin, TX, 78705, USA.8Department of Internal Medicine, Dell Medical School, 1501 Red River St, Austin, TX, 78701, USA.9Metro Health Medical Center, Case Western Reserve University School of Medicine, Health Education Campus, 9501 Euclid Ave, Cleveland, OH, 44106, USA.10Division of Cardiology, Stanford University, 870 Quarry Road Extension, Stanford, CA, 94305, USA.11Department of Biomedical Engineering, University of Texas at Austin, 107 W Dean Keeton St, Austin, TX, 78712, USA.12Interventional Electrophysiology, SCRIPPS Green Hospital, San Diego, CA, 92037, USA.13Icahn School of Medicine at Mount Sinai, 1 Gustave L. Levy Pl, New York, NY, 10029, USA.
A novel QDOT MICRO (Biosense Webster, Inc., Irvine, CA) catheter with optimized temperature control and microelectrodes was designed to incorporate real-time temperature sensing with contact force detection and microelectrodes to streamline ablation workflow. The QDOT-MICRO feasibility study evaluated the workflow, performance, and safety of temperature-controlled catheter ablation in patients with symptomatic paroxysmal atrial fibrillation with conventional ablation setting.
This was a non-randomized, single-arm, first-in-human study. The primary outcome was pulmonary vein isolation (PVI), confirmed by entrance block after adenosine and/or isoproterenol challenge. Safety outcomes included incidences of early-onset primary adverse events (AEs) and serious adverse device effects (SADEs). Device performance was evaluated via physician survey.
All evaluated patients (n = 42) displayed 100% PVI. Two primary AEs (4.8%) were reported: 1 pericarditis and 1 vascular pseudoaneurysm. An additional SADE of localized infection was reported in 1 patient. No stroke, patient deaths, or other unanticipated AEs were reported. Average power delivered was 32.1±4.1 W, with a mean temperature of 40.8°C±1.6°C. Mean procedure (including 20-minute wait), fluoroscopy, and radiofrequency application times were 129.8, 6.7, and 34.0 minutes, respectively. On device performance, physicians reported overall satisfactory performance with the new catheter, with highest scores for satisfaction and usefulness of the temperature indicator.
Initial clinical experience with the novel catheter showed 100% acute PVI success and acceptable safety and device performance in temperature-controlled ablation mode. There were no deaths, stroke, or unanticipated AEs. Fluoroscopy and procedural times were short and similar or better than reported for prior generation catheters.
Key Words : Atrial fibrillation, Workflow, Catheter ablation, Pulmonary vein isolation, Arrhythmia.
Tom De Potter, MD
OLV Hospital
Cardiology Department
Moorselbaan 164
9300 Aalst, Belgium
Catheter ablation is a well-recognized treatment option for paroxysmal atrial fibrillation (PAF) 1, and complete electrical isolation of the pulmonary veins (PVI) from the left atrium (LA) is recommended for all atrial fibrillation (AF) ablation procedures 2. Open-irrigated radiofrequency (RF) catheters are the most widely used for PAF ablation. More recently, RF catheters with real-time, contact force (CF)–sensing capability have become available, and studies have shown that these CF-sensing catheters improve procedural efficiencies and long-term success 3,4. A newer iteration of the CF-sensing catheter that includes a 56-hole porous tip (versus 6 larger holes), permitting PVI while delivering a reduced volume of fluid 5.
However, it has been reported that uniform cooling from the porous tip resulted in attenuation of temperature change within the catheter tip, even with increasing power levels 6. Furthermore, the temperature feedback, detected by a single thermocouple in the middle of the tip, is very poor.
A novel catheter with optimized temperature control and microelectrodes [Figure 1A] was designed to potentially enhance the safety and effectiveness of the currently available, porous tip, CF-sensing catheter by incorporating real-time temperature sensing with CF and microelectrodes to both adequately cool the catheter tip and retain optimal sensing of tissue temperature. The addition of a multi-channel RF generator and novel algorithm allows for temperature-controlled ablation by adjusting the irrigation rate and power based on temperature feedback ([Figure 1B]-[Figure 1E]). Microelectrodes were incorporated to enhance the quality of the near-field electrogram signals ([Figure 1A] and [Figure 1B]). Higher signal resolution gives the operator the ability to monitor signal attenuation during mapping and ablation while aiding in the identification of non-ablated tissue. The real-time temperatures recorded by the thermocouples are displayed graphically [Figure 1C]. A proprietary add-on software module was designed for the study to display and record electrocardiogram (ECG) results, temperature, location, and CF information.
Figure 1. (A) The QDOT MICRO catheter with 3 distal microelectrodes and 6 thermocouples; (B) visual display of the enhanced ECG signal attenuation obtained by Microelectrodes (Mic1, Mic2, and Mic3) in comparison to the traditional M1-M2 electrodes and the catheter’s associated location, as shown with CARTO3 integration; (C) real-time visual display of the temperatures recorded by the catheter; (D) temperature-control QMODE module algorithm with RF power ≤35 W; and (E) temperature-control QMODE module algorithm with RF power >35 W.
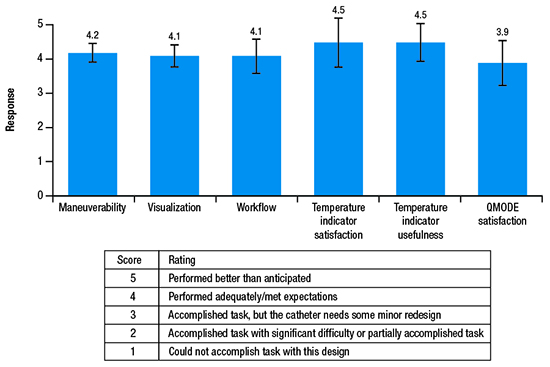
ECG, electrocardiogram; RF, radiofrequency.
The initial clinical experience with this catheter for PVI using a very high power, short duration (vHPSD) workflow (90 W, 4 seconds) has been described previously 7. The current QDOT-MICRO (Biosense Webster, Inc., Irvine, CA) workflow feasibility study was designed primarily to evaluate the workflow, acute success, device performance, and safety of the new catheter during standard electrophysiology mapping and PAF ablation 3 months post-procedure, using a standard ablation power setting (1W-45W) in a temperature-controlled mode.
This was a prospective, non-randomized, multi-center, clinical investigation performed in 6 clinics in Europe to evaluate the workflow and acute performance of the QDOT MICRO catheter in PAF ablation with temperature-controlled mode in the standard power setting. This temperature-controlled mode, known as the QMODE algorithm, automatically adjusts the irrigation flow rate between low flow (4 mL/min) to high flow (15 mL/min) to reach and maintain the set maximum power without exceeding the set target temperature. The maximum allowed power at the LA roof, LA ridge, and locations anterior and inferior to the PVs were directed not to exceed 45 W. For the LA posterior wall and areas close to the esophagus, ablation was directed to begin at ≤25 W. The utilization of an appropriate strategy (i.e., esophageal temperature probe, esophageal visualization, and/or esophageal displacement) to reduce esophageal injury was also required. Outcomes were assessed at baseline, at discharge, at the time of the ablation procedure, and at 7 days and 3 months post-procedure.
The study was conducted in accordance with the Declaration of Helsinki with E6 of the International Conference on Harmonisation Good Clinical Practices, ISO 14155-2011, and all applicable local and federal regulations. The study was also approved by the ethics committees in all participating countries. All study patients provided written informed consent.
Eligible patients were required to be ≥18 years of age and candidates for catheter ablation of symptomatic PAF. Patients were excluded if they had AF secondary to electrolyte imbalance, thyroid disease, or reversible or non-cardiac cause; previous ablation for AF; persistent AF; left atrial thrombus; carotid stenting or endarterectomy; LA size >50 mm; left ventricular ejection fraction <40%; uncontrolled heart failure or New York Heart Association function class III and IV; unstable angina; history of blood clotting or bleeding abnormalities or contraindication to anticoagulation; a thromboembolic event in the past 12 months; percutaneous coronary intervention for myocardial infarction in the past 3 months; cardiac surgery in conjunction with valve surgery or any valvular cardiac surgical/percutaneous procedure in the past 6 months; or expected cardiac transplantation or other cardiac surgery in the next 6 months.
In phase 1 of the study, 8 patients were enrolled. A lower electrode temperature response than anticipated, which may potentially result in less effective ablation lesions in certain ablation locations, was identified. Consequently, enrollment in the study was temporarily suspended while the design of the catheter was modified and the generator software was updated. Additional preclinical work was performed prior to subsequent enrollment to ensure that the anticipated temperature response was recorded by the electrodes. The modified device was used to perform the ablation procedure for 43 patients in phase 2 of the study.
Treatment with uninterrupted systemic anticoagulation therapy was required for at least 3 weeks prior to study treatment. During the ablation procedure, heparin was administered to achieve an activated clotting time of ≥325 seconds. Ablation was performed with the novel catheter under the temperature-controlled mode. Irrigation was automatically increased to a high flow rate up to 2 seconds before the onset through 5 seconds after termination of RF energy delivery.
Recommended temperature ranges and RF power settings were as follows: RF power range, 15 W to 45 W; target temperature setting, 47°C (range, 45°C-50°C) for ≤35 W or 42°C (range, 40°C-45°C) for >35 W; default temperature cutoff for the generator, 55°C; and default ablation time, up to 60 seconds. If the temperature increased rapidly, the RF application was stopped immediately. Esophageal monitoring was required to minimize the risk of esophageal injury and was conducted for 50 patients (temperature probe for 36, barium contrast for 9, and intracardiac echocardiography for 5).
A circumferential anatomical and endocardial signals approach was used to isolate all PVs. To minimize the risk of PV stenosis, RF applications were recommended to be ≥1 cm to 2 cm outside the PV ostia to isolate the left and right PVs. In the event of spontaneous or induced AF and/or atrial flutter, additional RF lesions outside of the PV ostia were placed at the discretion of the investigator. PVI via entrance block was assessed by Lasso or PentaRay catheters (Biosense Webster, Inc., Irvine, CA).
After a required 20-minute waiting period, adenosine or isoproterenol was used to confirm PVI and rule out dormant conduction. Oral anticoagulation therapy was strongly recommended for 2 months following ablation. Thereafter, patients were advised to receive anticoagulation therapy in accordance with the 2017 Heart Rhythm Society consensus statement 8. The administration of antiarrhythmic drugs after ablation was based on the treating physician’s discretion.
The primary effectiveness outcome was acute success, defined as confirmed entrance block in all targeted PVs via adenosine and/or isoproterenol challenge.
The main secondary endpoint was safety assessment, as defined by the number of early-onset primary adverse events (PAEs) within 7 days of catheter ablation. In this study, PAEs were defined as death, atrioesophageal fistula (including events >7 days post-procedure), cardiac tamponade/perforation, myocardial infarction, stroke/cerebrovascular accident, thromboembolism, transient ischemic attack, diaphragmatic paralysis, pneumothorax, heart block, PV stenosis (including events >7 days post-procedure), pulmonary edema (respiratory insufficiency), vagal nerve injury, pericarditis, major vascular access, and complication/bleeding. Additional serious adverse device effects (SADEs) over up to 3 months follow-up were also recorded.
Procedural endpoints included a mandatory ECG assessment at each clinical follow-up visit; rate of touch-up RF application, defined as RF applications for re-isolation of targeted PVs post adenosine and/or isoproterenol; and procedural characteristics. Device performance was assessed by a physician survey with a ranking system scoring from 1 to 5 (see table in [Figure 2]) on questions on maneuverability, visualization, workflow, temperature satisfaction, temperature indicator usefulness, and algorithm modality satisfaction.
Figure 2. Mean ± SD responses (possible score, 1-5) to the physician’s device performance survey.
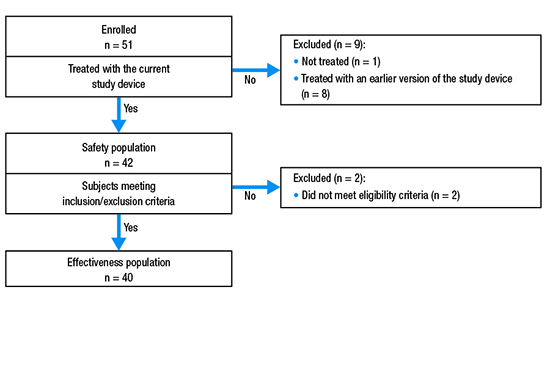
SD, standard deviation.
As this was a feasibility study, no hypothesis was tested, and all data are presented as descriptive statistics, including the number and percentage of patients who reached acute success. A total of 50 evaluable patients were deemed sufficient to characterize the workflow and acute outcomes of the study.
The effectiveness population included all enrolled patients who met all eligibility criteria and underwent ablation with the study catheter. The safety population included all enrolled patients who had the investigational device inserted, regardless of whether RF energy was delivered.
Demographics and baseline characteristics
Patients were enrolled between September 28, 2016 and April 26, 2018. A total of 51 patients were originally enrolled; however, after 8 patients were treated, the device was modified and those patients were excluded from analyses of efficacy and safety. Therefore, a total of 43 patients were enrolled in the study using the updated version of the device. The safety population included 42 patients who had the investigational device inserted (1 patient did not check in for their scheduled ablation procedure). Two patients who underwent ablation were later excluded from the effectiveness population (n = 40) based on failing eligibility criteria [Figure 3].
Figure 3. Patient disposition.
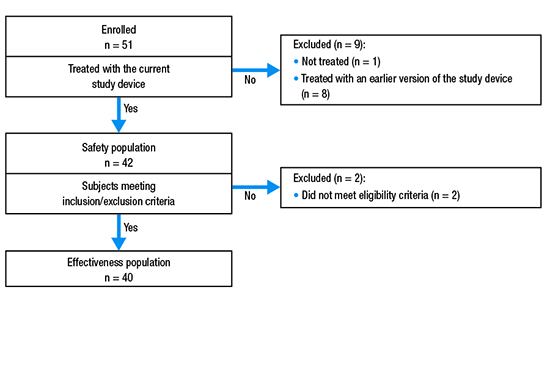
The majority of patients were male (62.8% [27/43]) and relatively young (mean ± standard deviation [SD] age = 60.3 ± 12.6 years; [Table 1]). The most common medical comorbidities were systemic hypertension (55.8%) and coronary disease (16.3%; [Table 2]).
Table 1. Baseline Patient Characteristics
Characteristics |
Enrolled population(N = 43)* |
Age, years, mean ± SD |
60.3 ± 12.6 |
Sex, male, n (%) |
27 (62.8%) |
Patient history |
- |
AF duration, months, median (Q1, Q3) |
24 (10.0, 59.0) |
Atrial flutter, n (%) |
6 (14.0%) |
Systemic hypertension, n (%) |
24 (55.8%) |
Diabetes, n (%) |
2 (4.7%) |
Coronary disease, n (%) |
7 (16.3%) |
Prior thromboembolic events/TIA, n (%) |
4 (9.3%)/1 (2.3%) |
Hyperthyroidism, n (%) |
2 (4.7%) |
CHA2DS2-VASc score, mean ± SD |
1.8 ± 1.31 |
Congestive heart failure, n (%) |
1 (2.3%) |
Failed anti-arrhythmic drug class, n (%) |
38 (88.4%) |
LVEF (%), mean ± SD (min, max) |
60.6 ± 5.38 (50.0, 73.0) |
LA dimension, mm, mean ± SD (min, max) |
LA dimension, mm, mean ± SD (min, max) |
AF, atrial fibrillation; LA, left atrium; LVEF, left ventricular ejection fraction; Q, quartile; SD, standard deviation; TIA, transient ischemic attack.
Procedural characteristics
Across 42 treated patients, the mean ± SD total procedure time, defined as the time from first puncture until the time the catheter was removed and including 20-minute wait times, was 129.8 minutes ± 27.2 minutes ([Figure 4]; [Table 2]). Average mapping, fluoroscopy, total ablation, and RF application times are summarized in [Table 2].
Figure 4. (A) Mean procedure,* fluoroscopy, ablation,† and RF application duration times; and (B) fluid delivery via the study catheter in the QDOT-MICRO and SMART SF studies.5

PV, pulmonary vein; RF, radiofrequency.
On average, 62 RF applications were performed per procedure (from generator data), with an average 32-second RF duration per application [Table 2]. The number of applications to the left and right PVs was similar.
Table 2. Procedural Characteristics
Variable, mean ± SD (Q1, Q3) |
Safety population (N = 42) |
Total procedure time, minutes* |
129.8 ± 27.2 (106.0, 153.0) |
Total mapping time, minutes |
9.5 ± 7.6 (3.0, 13.0) |
Total ablation time, minutes† |
67.2 ± 22.4 (47.0, 81.0) |
Total RF application duration, minutes‡ |
34.0 ± 10.8 (27.3, 36.5) |
Total fluoroscopy time, minutes |
6.7 ± 9.1 (2.4, 6.1) |
Fluid delivered via study catheter(s), mL |
572.5 ± 296.1 (400.0, 750.0) |
Number of RF applications§ |
62.5 ± 19.9 (49.0, 73.0) |
Total RF duration per application, seconds§ |
31.5 ± 14.3 (21.4, 40.0) |
Maximum temperature, °C§ |
51.4 ± 1.93 (50.4, 52.5) |
Average power, W§ |
32.1 ± 4.13 (29.6, 33.3) |
Q, quartile; RF, radiofrequency; SD, standard deviation.
Average power delivered during the workflow, maximum temperature measured by the catheter, and fluid delivered via the catheter are summarized in [Table 2]. The most commonly selected CF working ranges were 3 g to 40 g (35.7% [15/42]) and 5 g to 30 g (35.7% [15/42]), and the average CF applied was 15.3 g ± 4.0 g.
Of the 40 patients in the effectiveness population, 39 were evaluated via adenosine and/or isoproterenol challenge after entrance block confirmation; 1 patient did not have the adenosine challenge and was removed from the primary performance analysis. Entrance block in all targeted PVs was achieved in all 39 (100%) treated and evaluated patients per the endpoint definition. Entrance block was confirmed at the end of the procedure for all PV targets independently of adenosine and/or isoproterenol challenge (n = 40). At the 3-month follow-up visit, 92.7% (38/41) of patients with an ECG were in sinus rhythm and did not report any arrhythmia recurrence.
Adverse events (AEs) are summarized in [Table 3]. Of the 42 patients in the safety population, 2 (4.8%) experienced PAEs: 1 pericarditis and 1 vascular pseudoaneurysm. Both events resolved without sequelae and were considered to be related to the procedure but not the device. There was an additional SADE of localized infection that was considered to be probably related to the procedure but not the device and resolved without sequelae. No SADEs were reported beyond 7 days after the investigational procedure. There were no strokes, device-related deaths, or unanticipated serious AEs.
Table 3. Summary of Safety
Event |
Classification |
Severity |
Causality |
Outcome |
Vascular pseudoaneurysm |
PAE/SADE |
Mild |
Definitely procedure related |
Resolved without sequelae |
Pericarditis |
PAE/SADE |
Moderate |
Definitely procedure related |
Resolved without sequelae |
Localized infection |
SADE |
Moderate |
Probably procedure related |
Resolved without sequelae |
PAE, primary adverse event; SADE, serious adverse device effect.
Rate of touch-up RF application and repeat ablation
Eight patients required touch-up application after adenosine challenge, the majority (n = 6) of which were at the right PV. PV touch-up ablation sites included 1 site in the posterior left PV, 1 in the anterior right PV (RPV), 6 in the posterior RPV, and 1 in the ridge RPV. No patients had a repeat ablation procedure during the 3-month follow-up period.
Physician surveys on device performance
Results of the physician survey indicated that the catheter performed adequately and met expectations in all aspects of device performance, including catheter maneuverability, visualization, interaction with other devices in the current ablation workflow, usefulness of/satisfaction with the temperature indicator, and satisfaction with the novel algorithm [Figure 2]. The usefulness of and satisfaction with the temperature indicator each received the highest mean scores of 4.5 out of 5. Specifically, operators provided feedback that the temperature indicator was useful in confirming heating (mean, 4.4/5) and providing confidence in catheter stability (mean, 4.5/5). The operators also indicated a satisfactory learning curve for the catheter in terms of maneuverability (mean, 4.4/5).
The results of our study demonstrate that the new catheter with automatic irrigation modulation allows for temperature-controlled ablation in the standard power setting and achieved acute success in all treated patients. The low rates of PAEs (4.8%) suggested an acceptable initial safety profile, with similar or better procedural efficiency than reported in previous studies [Figure 4] 3,5,9.
The complication rate reported in this initial study is consistent with rates from standard power-controlled ablation 8. In addition, all complications (vascular access complication, pericarditis, infection) reported were related to the procedure and unrelated to the device. The previous porous tip catheter was designed to provide more uniform cooling of the electrode tip for more efficient RF delivery and reduced fluid delivery 10. However, it has been suggested that the catheter tip maintains a constant temperature throughout the RF application and lacks the usual temperature feedback commonly used to monitor safe and efficient RF delivery 11. The novel catheter investigated in this study incorporates a number of surface thermocouples (6) with proximity to the cardiac tissues, which enables enhanced and localized temperature measurement. This feature allows a marked reduction in the risk of char formation; indeed, even when the tip pushes on the tissue, closing some of the irrigation holes, very accurate temperature feedback protects against blood and tissue overheating. In addition, the ability of the novel proprietary algorithm, which allows for modulation of the irrigation and power to maintain target temperatures, may have contributed to the observed low PAE rate in the temperature-controlled ablation mode. Other than the 3 reported PAEs/SADEs, there were no reports of serious AEs of stroke, thromboembolism, or other unanticipated AEs.
In addition to safety, the combination of improved temperature sensitivity and availability of the temperature graphic indicator may reduce the number of RF applications, as well as ablation and procedure times. The physicians’ survey suggested that the temperature indicator [Figure 1B] provides useful feedback in confirming heating and catheter stability, potentially facilitating workflow efficiencies. In fact, procedure, fluoroscopy, ablation, and RF times reported in this study were short, generally shorter than in a previous study (SMART SF) using the previous porous tip catheter 5 ([Figure 4]; procedure time, −28%; fluoroscopy time, −64%; ablation time, −36%; RF ablation time, −31%). Of note, the fluoroscopy time achieved in the current study was minimal. The study protocol did not stipulate a specific workflow to target lowering the fluoroscopy time, and all operators had extensive prior experience in RF ablation. The quality of the endocavitary signals were improved by the microelectrodes [Figure 1], likely contributing to the efficiency of the procedure. Specifically, the microelectrodes, which selectively record local potentials, allow for a reduction in RF time by displaying the disappearance of the potential in real time, thus avoiding unnecessary RF delivery to far-field potentials. Taken together, the differences in procedural efficiencies between the current and prior studies are therefore likely attributable, at least in part, to the new catheter design and algorithm. For example, while the operators may have to vary CF and adjust power using existing catheters, the novel algorithm automatically titrates power and/or adjusts irrigation based on a predefined target temperature (47°C for ≤35 W or 42°C for >35 W), thereby simplifying the ablation workflow and leading to the gain in efficiencies.
Ultimately, the temperature-sensing capability reintroduces verification of the end goal of RF ablation―tissue heating―by measuring the temperature at the tip-tissue interface, a capability long held by conventional RF catheters that was lost with the introduction of irrigated tip catheters. In addition, the incorporation of the 6 thermocouple temperature sensors to the electrode tip of this catheter was associated with lower flow rates than previous catheter designs and led to a reduction in total fluid volume delivered. This temperature indication may therefore help improve long-term effectiveness. As previously shown, catheter stability is important in optimizing the long-term success rate 12. The ability to consistently reach the target temperature and to maintain desired power levels at a safe temperature could be key to creating effective and durable lesions. The effectiveness is also increased by the ability of this catheter to create deep lesions when needed, such as on the anterior wall of the left and right PVs. New and emerging technologies, such as bipolar RF, high-power RF, and electroporation, are more limited in producing deep lesions. Since this study was limited to a 3-month follow-up, this hypothesis will need to be tested in future studies with longer follow-up.
Although not evaluated in this study, the novel catheter is capable of ablating in vHPSD mode during the same ablation procedure. Clinical study using this catheter for vHPSD (90 W, 4 seconds) ablation showed a similarly low fluoroscopy time of 6.6 minutes and an even lower procedure time of 105.2 minutes ± 24.7 minutes in PVI procedures 7. However, unlike the QDOT-FAST study 7, the current study used standard power ablation settings, with a mean power of 32.1 W ± 4.1 W and mean RF duration of 31.5 seconds ± 14.3 seconds. In addition, the target temperature for the current study was lower compared to the QDOT-FAST study (40°C-50°C versus 60°C).
The PAE rate associated with the vHPSD ablation mode (3.8%) was similar to that with the standard power ablation mode in the current study. The ability to choose between vHPSD ablation for PVI or standard temperature-controlled ablation for PVI or extra PVI ablation, as needed, is an added clinical benefit of the new catheter/algorithm that allows for versatility in adapting to different ablation workflows. Other temperature-sensing irrigated catheters that are currently under clinical investigation only allow a maximum power of up to 50 W but have shown similar short, average RF application times of 26.3 minutes ± 5.2 minutes 13.
There are several limitations to this study. The single-arm, non-randomized design precluded direct comparisons to other devices. Additionally, the study results and conclusion are based on descriptive analyses for a relatively small sample size of patients, and follow-up was performed only up to 3 months. Larger studies with a formal hypothesis and statistical power calculations are needed to confirm the safety and long-term effectiveness of the new catheter.
The novel catheter with a microelectrode system and enhanced temperature sensing reintroduced temperature-controlled ablation to a porous-tip irrigated catheter, with an acceptable safety profile. Initial clinical experience showed high acute success, with efficient workflow and low fluoroscopy exposure time.