Cardiac Neuroanatomy for the Cardiac Electrophysiologist
Peter Hanna1,2, Jeffrey L. Ardell1,2, Kalyanam Shivkumar1,2
1University of California Los Angeles (UCLA) Cardiac Arrhythmia Center and Neurocardiology Research Program of Excellence, Department of Medicine, UCLA, Los Angeles, CA.2UCLA Molecular, Cellular & Integrative Physiology Program, UCLA, Los Angeles, CA.
The cardiac neuraxis is integral to cardiac physiology, and its dysregulation is implicated in cardiovascular disease. Neuromodulatory therapies are being developed that target the cardiac autonomic nervous system (ANS) to treat cardiac pathophysiology. An appreciation of the cardiac neuroanatomy is a prerequisite for development of such targeted therapies. Here, we provide a review of the current understanding of the cardiac ANS. The parasympathetic and sympathetic nervous system are composed of higher order cortical centers, brainstem, spinal cord, intrathoracic extracardiac ganglia and intrinsic cardiac ganglia. A series of interacting feedback loops mediates reflex pathways to exert control over the cardiac conduction system and contractile tissue. Further exploration of this complex regulatory system promises to yield neuroscience-based therapeutics for cardiac disease.
Key Words : Atrial Fibrillation, Neuroanatomy, Electrophysiologist.
Correspondence to: Kalyanam Shivkumar, MD PhD
100 UCLA Medical Plaza, Suite 660
Los Angeles, CA 90095
The autonomic nervous system (ANS) plays a critical role in many facets of cardiac physiology (e.g., modulation of chronotropy, dromotropy, inotropy, and lusitropy) 1. Historically, the nature of this role has been fervently debated, particularly in the case of cardiac conduction. The Italian physiologist Giovanni Borelli in the 17th century posited the neurogenic origin of the heartbeat, and this notion was widely accepted until Sir Walter Gaskell’s experimental work of the late 19th century propelled myogenic theory to the forefront. Furthermore, prior to the advent of direct cardiac interventions such as coronary revascularization for coronary artery disease, nerves were transected to treat angina 2. In recent years, our understanding of the exquisite control of the ANS in cardiac physiology has significantly improved, and the ANS has increasingly been targeted in cardiovascular diseases including arrhythmia, myocardial infarction and heart failure 3-6.
The ANS has traditionally been characterized as composed of two opposing limbs, the parasympathetic and sympathetic nervous systems, under the influence of the central nervous system (CNS). More extensive study, however, has demonstrated that a series of reflex pathways integrate afferent (sensory) and efferent (motor) information in a complex manner to regulate cardiac excitability and mechanical function Figure 1. These reflex pathways involve a cardioneural hierarchy that includes the intrinsic cardiac ganglia, extracardiac intrathoracic ganglia, spinal cord, brainstem, and higher cortical centers. Much of the pharmacologic therapy for heart failure and arrhythmia impact the neurohormonal axis, and neuromodulatory treatment modalities that are currently being explored in pre-clinical and clinical studies include vagal nerve stimulation (VNS), cardiac sympathetic denervation, renal denervation, spinal cord stimulation, baroreflex activation therapy, neurotoxin (botulinum toxin) injection and tragus stimulation 4,5. However, these interventions have had varying levels of success. Further understanding of the innervation pathways to the heart and a departure from an overly simplistic view of the sympathetic and parasympathetic nervous systems will promote development of new or improved targeted neuromodulatory therapeutics as alternatives to conventional approaches.
Figure 1. Neural control of the heart.

The cardiac autonomic nervous system consists of interacting feedback loops involving afferent (blue), efferent (red) and local circuit (blue and grey) neurons at the intracardiac (level 1), intrathoracic extracardiac (level 2) and central nervous system (level 3) levels. SG, stellate ganglion; DRG, dorsal root ganglion; ICNS, intrinsic cardiac nervous system. (Adapted from Shivkumar et al., 2016)
The notion of the brain-heart axis typically evokes thoughts of stress-induced cardiomyopathy or ‘broken heart syndrome’. Recent data has shown that lower socioeconomic status in patients with stress-induced cardiomyopathy is associated with increased amygdala activity on functional MRI and worse survival 7. Psychological stress is also associated with arrhythmia, as has been demonstrated in patients with implantable cardioverter-defibrillators or children playing electronic games 8,9. However, the importance of this axis is more pervasive, as population studies have demonstrated the link between stress and cardiovascular disease. Increases in cardiovascular events shortly after catastrophic events such as the Northridge earthquake in California in 1994 or the September 11th, 2001 attacks or sporting events such as the 2006 World Cup highlight the importance of the brain-heart axis 10-12. Cardiac sequalae of CNS insults such as stroke and seizure are multiple and include electrocardiographic changes and arrhythmias such as atrial fibrillation, atrioventricular (AV) block, sudden cardiac death and changes in heart rate variability, a surrogate marker of autonomic tone 13-20.
Pre-clinical models and human studies have helped discern important loci within the central nervous system that regulate cardiac function Figure 2. In rats, sites implicated in cardiac chronotropic control were found in the insular cortex 21. Neural tract tracing of the cardiac inputs of the vagus nerves has elucidated that preganglionic parasympathetic neurons origin in the brainstem within in the dorsal motor nucleus and nucleus ambiguus of the medulla oblongata and an intermediate zone between these two structures 22-26 Specifically, functional studies of the cat brainstem has demonstrated that neurons of the dorsal motor nucleus control ventricular contractility while those of the nucleus ambiguus regulate heart rate 27. A study in human patients demonstrated that strokes impacting the right insular cortex were associated with abnormalities in heart rate variability and increased incidence of complex arrhythmias 28. Functional MRI has also demonstrated that when the anterior cingulate cortex is impacted in a stroke, autonomic effector function is impacted as demonstrated by changes in heart rate variability 18.
Figure 2. Parasympathetic innervation of the heart.

Schematic demonstrating efferent (solid black line) and afferent (dashed black line) pathways to and from the heart, respectively. Preganglionic parasympathetic motor neurons are mainly found in the nucleus ambiguous and the dorsal motor nucleus in the medulla oblongata. Neurons then project via long axons in the vagus nerve to the intrinsic cardiac neurons in the heart. Sensory information is transduced via afferent fibers with cell bodies housed in the nodose ganglia. These neurons synapse in the nucleus tractus solitarius. Interneurons are present between the nucleus tractus solitarius, dorsal motor nucleus and nucleus ambiguus. (Adapted from Rajendran et al., 2017.)
Neuroanatomy of parasympathetic and sympathetic nervous systems
To explore the functional control of the cardiac neuraxis, a firm understanding of the cardiac neuroanatomy is imperative. Myocardial innervation patterns across mammals, including humans, are conserved 29-33, and the cardiac ANS has been categorized into: (1) central; (2) intrathoracic extracardiac; and (3) intrinsic cardiac components Figure 1. The intrathoracic extrinsic cardiac nervous system connects the intrinsic cardiac nervous system at the level of the heart to the CNS and is composed of sensory nerves and parasympathetic and sympathetic motor components that exert opposing effects on cardiac electrical and mechanical properties.
Parasympathetic motor neurons
Preganglionic parasympathetic neurons originating in the brainstem as described above traverse the bilateral vagus nerves and multiple intrathoracic cardiopulmonary branches to synapse on efferent postganglionic parasympathetic neurons in the numerous intrinsic cardiac ganglia Figure 2 34,35. Interestingly, a small population of sympathetic fibers have also been found to be contained with the vagi 36. Preganglionic parasympathetic neurons release neurotransmitter acetylcholine to activate the postganglionic neuron via nicotinic and muscarinic cholinergic receptors. Postganglionic neurons in turn secrete acetylcholine to activate muscarinic receptors expressed in the myocardium and coronary vasculature to mediate changes in chronotropy, dromotropy, inotropy and lusitropy.
Sympathetic motor neurons
Sympathetic efferent preganglionic neurons originate in the brainstem reticular formation, including the ventrolateral medulla, and track via the intermediolateral cell column of the spinal cord Figure 3. The neurons exit the spinal cord via the bilateral C7-T6 ventral rami to postganglionic sympathetic neurons in the superior cervical, middle cervical, cervicothoracic (stellate), and mediastinal ganglia 37,38. The preganglionic neurons send signals via acetylcholine, which binds nicotinic cholinergic receptors on the postganglionic neurons. These postganglionic neurons project axons via several cardiopulmonary nerves to the myocardium and limited populations of intrinsic cardiac adrenergic neurons. These neurons release neurotransmitter norepinephrine, which binds α- and β-adrenergic receptors expressed on the myocardium and coronary vasculature to increase chronotropy, inotropy and vasoconstriction.
Figure 3. Sympathetic innervation of the heart.

Schematic demonstrating sympathetic efferent (solid black lines) and afferent (dashed black lines) pathways to and from the heart. Preganglionic motor neurons originating in the brainstem reticular formation (BSRF), including the rostral ventrolateral medulla, descend the intermediolateral lateral column of the spinal cord and exit via ventral rami to synapse on postganglionic neurons in ganglia of the cervicothoracic paravertebral chain. The postganglionic neurons primarily synapse directly on the heart. Afferent information is conveyed via neurons whose cell bodies are found in the dorsal root ganglia (DRG) and centripetal axons project to the dorsal horn of the spinal cord. Second-order neurons project from the dorsal horn to the thalamus via the spinothalamic tract. (Adapted from Rajendran et al., 2017.)
Parasympathetic-sympathetic interactions
Challenging the dogma that the parasympathetic and sympathetic nervous system act as independent regulators over cardiac function is the body of literature describing interactions between the parasympathetic and sympathetic nervous system in the cardiac neural hierarchy. For example, animal studies have shown that ablation of the RAGP significantly decreases VNS-induced bradycardia but does not impact the indirect influence on stellate ganglia stimulation-induced tachycardia during concurrent VNS 39. Similarly, ablation of the RAGP and IVC-IA GP mitigated VNS-mediated slowing of AV conduction, but VNS still reduced sympathetic stimulation-induced increases in AV conduction. Furthermore, transecting the bilateral vagi and stimulating the central end of the vagi still mediated effects on heart rate suggesting activation of vagal afferents still impacted central inputs to the heart 40.
Cardiac afferents provide beat-to-beat sensory information of cardiac function and microenvironment to the neuraxis, and additional information is conveyed by arterial mechano- and chemoreceptors Figure 2. Around 80% of the fibers in the vagus nerve are afferents and travel via the nodose ganglia to transduce sensory information to the nucleus tractus solitarius in the brainstem 41-43. Cell bodies of afferent neurons in ‘sympathetic’ fibers are in the C7-T4 dorsal root ganglia and synapse on second-order neurons that project to the thalamus via the spinothalamic tract 44,45. Sensory neurons have also been identified in the intrinsic cardiac ganglia 46,47. The processing of afferent information at multiple levels, including the intrinsic cardiac nervous system, extracardiac intrathoracic ganglia, spinal cord, brain stem, and higher centers, provides an elegant mechanism of interacting feedback loops that modulate efferent cardiomotor (sympathetic and parasympathetic) signals for maintaining normal rhythm and life-sustaining circulation.
The intrinsic cardiac nervous system
Analogous to the enteric nervous system of the digestive tract, the intrinsic cardiac neurons are found in intramural ganglia and in epicardial fat pads Figure 4 48,49. While these neuronal somata were identified early in the last century, the location and connections of these neurons remained poorly understood 50. Subsequent light and electron microscopy studies identified these structures in the human heart 49. It is now known that the intrinsic cardiac ganglionated plexi contain a distributed network of afferent, motor (parasympathetic and sympathetic) and interconnecting (local circuit) neurons 51. The ICNS, under the influence of inputs from the brainstem, spinal cord and intrathoracic sympathetic ganglia, is thought to serve as a final coordinator of regional reflexes. Taken together, this neural network at the level of the heart is thought to play an important role in modulating cardio-cardiac reflexes.
Figure 4. Intrinsic cardiac nervous system is comprised of several ganglionated plexi (GPs) that are primarily found the heart hilum.
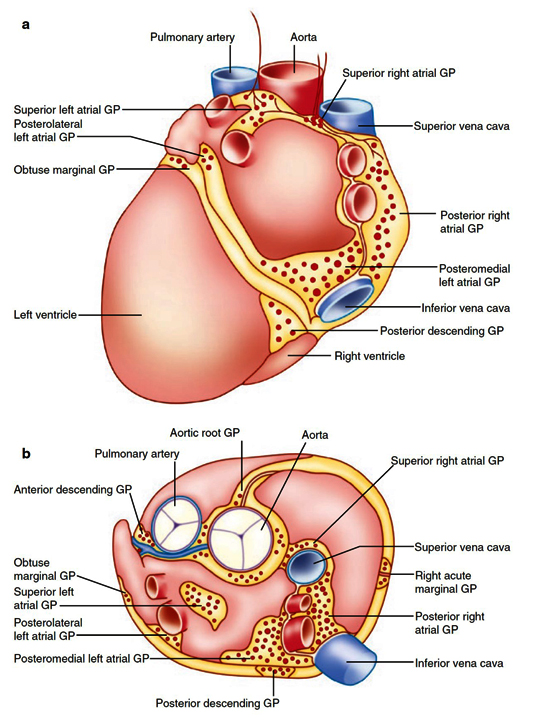
a Left posterior oblique view of the heart.
b Superior view of the heart. (Adapted from Rajendran et al., 2017.).
While postganglionic neurons in individual ganglionated plexi (GPs) supply both atrial and ventricular tissues, certain GPs influence discrete cardiac regions. Anatomic dissections and functional studies in canines have demonstrated that postganglionic vagal neurons to the sinoatrial (SA) node are found predominantly in a GP next to the right pulmonary vein-atrial junction known as the right atrial ganglionated plexus (GP), while the postganglionic vagal neurons that influence the atrioventricular (AV) node are located predominantly in the region adjacent to the inferior vena cava-inferior left atrium junction 52. Sympathetic fibers from the right stellate ganglion course through the posterior atrial GP located between the superior vena cava and aorta, while parasympathetic neurons in this GP exerts a negative chronotropic effect on the SAN 53,54. Parasympathetic neurons in the ventral interventricular GP exert negative ionotropic effects on left ventricular contractility while sympathetic fibers also course through this region 55. However, while it may be tempting to construe that specific GPs (e.g. the RAGP) within the ICNS may be ablated to affect a particular cardiac region (e.g. the SA node), pleiotropic effects of the GPs on other aspects of cardiac function invite off-target effects if such an approach is adopted (Hanna et al., submitted).
Figure 5. Schematic of neural control and functional organization of the cardiac ANS.

Interacting feedback loops within the cardiac neuraxis exert control over the heart. Current neuromodulatory therapies and their sites of action are listed alongside the diagram. Aff, afferent; β1, β-adrenergic receptor; C, cervical; DRG, dorsal root ganglion; Gi, inhibitory G-protein; Gs, stimulatory G-protein; L, lumbar; LCN, local circuit neuron; M2, muscarinic receptor; T, thoracic. Adapted from Shivkumar et al. (2016).
The cardiac ANS regulates all aspects of cardiac electrical activity and mechanical function. Reflex pathways that span the cortex to the ICNS maintain sympathovagal balance. Crosstalk amongst these feedback loops across the neural hierarchy, mixed neuron populations within the ICNS and mixed nerves create a distributed network for autonomic control Figure 5. Multiple nexus points for current neurointerventions are found within these pathways. Further study of the cardiac neuroanatomy is necessary for the development of precise neuromodulatory therapeutics for cardiovascular disease.
- Shivkumar K, Ajijola OA, Anand I, Armour JA, Chen PS, Esler M, et al. Clinical neurocardiology defining the value of neuroscience‐based cardiovascular therapeutics. The Journal of physiology. 2016;594(14):3911-54.
- Birkett D, Apthorp G, Chamberlain D, Hayward G, Tuckwell E. Bilateral upper thoracic sympathectomy in angina pectoris: results in 52 cases. British medical journal. 1965;2(5455):187.
- White SK, Frohlich GM, Sado DM, Maestrini V, Fontana M, Treibel TA, et al. Remote ischemic conditioning reduces myocardial infarct size and edema in patients with ST-segment elevation myocardial infarction. JACC: Cardiovascular Interventions. 2015;8(1 Part B):178-88.
- Zhu C, Hanna P, Rajendran PS, Shivkumar K. Neuromodulation for Ventricular Tachycardia and Atrial Fibrillation: A Clinical Scenario-Based Review. JACC Clin Electrophysiol. 2019;5(8):881-96.
- Hanna P, Shivkumar K, Ardell JL. Calming the nervous heart: autonomic therapies in heart failure. Cardiac Failure Review. 2018;4(2):92.
- Stavrakis S, Kulkarni K, Singh JP, Katritsis DG, Armoundas AA. Autonomic Modulation of Cardiac Arrhythmias. JACC: Clinical Electrophysiology. 2020;6(5):467.
- Tawakol A, Osborne MT, Wang Y, Hammed B, Tung B, Patrich T, et al. Stress-associated neurobiological pathway linking socioeconomic disparities to cardiovascular disease. Journal of the American College of Cardiology. 2019;73(25):3243-55.
- Lampert R, Joska T, Burg MM, Batsford WP, McPherson CA, Jain D. Emotional and physical precipitants of ventricular arrhythmia. Circulation. 2002;106(14):1800-5.
- Lawley CM, Skinner JR, Turner C. Syncope due to ventricular arrhythmia triggered by electronic gaming. New England Journal of Medicine. 2019;381(12):1180-1.
- Leor J, Poole WK, Kloner RA. Sudden cardiac death triggered by an earthquake. New England Journal of Medicine. 1996;334(7):413-9.
- Steinberg JS, Arshad A, Kowalski M, Kukar A, Suma V, Vloka M, et al. Increased incidence of life-threatening ventricular arrhythmias in implantable defibrillator patients after the World Trade Center attack. Journal of the American College of Cardiology. 2004;44(6):1261-4.
- Wilbert-Lampen U, Leistner D, Greven S, Pohl T, Sper S, Völker C, et al. Cardiovascular events during World Cup soccer. New England Journal of Medicine. 2008;358(5):475-83.
- Burch G, MEYERS R, Abildskov J. A new electrocardiographic pattern observed in cerebrovascular accidents. Circulation. 1954;9(5):719-23.
- Wong TC, Cooper ES. Atrial fibrillation with ventricular slowing in a patient with spontaneous subarachnoid hemorrhage. The American journal of cardiology. 1969;23(3):473-7.
- Mathew NT, Taori G, Mathai K, Chandy J. Atrial fibrillation associated with seizure in a case of frontal meningioma. Neurology. 1970;20(7):725-.
- Myers MG, Norris JW, Hachinski V, Weingert M, Sole M. Cardiac sequelae of acute stroke. Stroke. 1982;13(6):838-42.
- Tokgözoglu SL, Batur MK, Topçuoglu MA, Saribas O, Kes S, Oto A. Effects of stroke localization on cardiac autonomic balance and sudden death. Stroke. 1999;30(7):1307-11.
- Critchley HD, Mathias CJ, Josephs O, O'Doherty J, Zanini S, Dewar BK, et al. Human cingulate cortex and autonomic control: converging neuroimaging and clinical evidence. Brain. 2003;126(10):2139-52.
- Goldman AM. Mechanisms of sudden unexplained death in epilepsy. Current opinion in neurology. 2015;28(2):166.
- van der Lende M, Surges R, Sander JW, Thijs RD. Cardiac arrhythmias during or after epileptic seizures. Journal of Neurology, Neurosurgery & Psychiatry. 2016;87(1):69-74.
- Oppenheimer SM, Cechetto DF. Cardiac chronotropic organization of the rat insular cortex. Brain research. 1990;533(1):66-72.
- Ciriello J, Calaresu F. Distribution of vagal cardioinhibitory neurons in the medulla of the cat. American Journal of Physiology-Regulatory, Integrative and Comparative Physiology. 1980;238(1):R57-R64.
- Nosaka S, Yasunaga K, Tamai S. Vagal cardiac preganglionic neurons: distribution, cell types, and reflex discharges. American Journal of Physiology-Regulatory, Integrative and Comparative Physiology. 1982;243(1):R92-R8.
- Standish A, Enquist LW, Schwaber JS. Innervation of the heart and its central medullary origin defined by viral tracing. Science. 1994;263(5144):232-4.
- Standish A, Enquist LW, Escardo J, Schwaber J. Central neuronal circuit innervating the rat heart defined by transneuronal transport of pseudorabies virus. Journal of Neuroscience. 1995;15(3):1998-2012.
- Ai J, Epstein P, Gozal D, Yang B, Wurster R, Cheng Z. Morphology and topography of nucleus ambiguus projections to cardiac ganglia in rats and mice. Neuroscience. 2007;149(4):845-60.
- Geis GS, Wurster RD. Cardiac responses during stimulation of the dorsal motor nucleus and nucleus ambiguus in the cat. Circulation Research. 1980;46(5):606-11.
- Colivicchi F, Bassi A, Santini M, Caltagirone C. Cardiac autonomic derangement and arrhythmias in right-sided stroke with insular involvement. Stroke. 2004;35(9):2094-8.
- Kuntz A, MOREHOUSE A. Thoracic sympathetic cardiac nerves in man: Their relation to cervical sympathetic ganglionectomy. Archives of Surgery. 1930;20(4):607-13.
- Saccomanno G. The components of the upper thoracic sympathetic nerves. Journal of comparative Neurology. 1943;79(3):355-78.
- Mizeres NJ. The cardiac plexus in man. American Journal of Anatomy. 1963;112(2):141-51.
- RANDALL WC, WC R, JA A. Regional cardiac distribution of the sympathetic nerves. 1972.
- Janes RD, Brandys JC, Hopkins DA, Johnstone DE, Murphy DA, Armour JA. Anatomy of human extrinsic cardiac nerves and ganglia. The American journal of cardiology. 1986;57(4):299-309.
- Hopkins DA, Bieger D, de Vente J, Steinbusch HW. Vagal efferent projections: viscerotopy, neurochemistry and effects of vagotomy. Progress in brain research. 107: Elsevier; 1996. p. 79-96.
- Ardell JL, Rajendran PS, Nier HA, KenKnight BH, Armour JA. Central-peripheral neural network interactions evoked by vagus nerve stimulation: functional consequences on control of cardiac function. American Journal of Physiology-Heart and Circulatory Physiology. 2015;309(10):H1740-H52.
- Seki A, Green HR, Lee TD, Hong L, Tan J, Vinters HV, et al. Sympathetic nerve fibers in human cervical and thoracic vagus nerves. Heart Rhythm. 2014;11(8):1411-7.
- Hopkins DA, Armour JA. Localization of sympathetic postganglionic and parasympathetic preganglionic neurons which innervate different regions of the dog heart. Journal of Comparative Neurology. 1984;229(2):186-98.
- Kawashima T. The autonomic nervous system of the human heart with special reference to its origin, course, and peripheral distribution. Anatomy and embryology. 2005;209(6):425-38.
- McGuirt A, Schmacht D, Ardell J. Autonomic interactions for control of atrial rate are maintained after SA nodal parasympathectomy. American Journal of Physiology-Heart and Circulatory Physiology. 1997;272(6):H2525-H33.
- Rajendran PS, Challis RC, Fowlkes CC, Hanna P, Tompkins JD, Jordan MC, et al. Identification of peripheral neural circuits that regulate heart rate using optogenetic and viral vector strategies. Nature communications. 2019;10(1):1-13.
- Foley JO, DuBois FS. Quantitative studies of the vagus nerve in the cat: I. The ratio of sensory to motor fibers. The Journal of Nervous and Mental Disease. 1937;86(5):587.
- Hoffman HH, Schnitzlein HN. The numbers of nerve fibers in the vagus nerve of man. The Anatomical Record. 1961;139(3):429-35.
- Hopkins DA, Armour JA. Ganglionic distribution of afferent neurons innervating the canine heart and cardiopulmonary nerves. Journal of the autonomic nervous system. 1989;26(3):213-22.
- Brown A. Excitation of afferent cardiac sympathetic nerve fibres during myocardial ischaemia. The Journal of physiology. 1967;190(1):35.
- Vance W, Bowker R. Spinal origins of cardiac afferents from the region of the left anterior descending artery. Brain research. 1983;258(1):96-100.
- Ardell J, Butler C, Smith F, Hopkins D, Armour J. Activity of in vivo atrial and ventricular neurons in chronically decentralized canine hearts. American Journal of Physiology-Heart and Circulatory Physiology. 1991;260(3):H713-H21.
- Cheng Z, Powley TL, Schwaber JS, Doyle III FJ. Vagal afferent innervation of the atria of the rat heart reconstructed with confocal microscopy. Journal of Comparative Neurology. 1997;381(1):1-17.
- Yuan BX, Ardell JL, Hopkins DA, Losier AM, Armour JA. Gross and microscopic anatomy of the canine intrinsic cardiac nervous system. The Anatomical Record. 1994;239(1):75-87.
- Armour JA, Murphy DA, Yuan BX, MacDonald S, Hopkins DA. Gross and microscopic anatomy of the human intrinsic cardiac nervous system. The Anatomical Record: An Official Publication of the American Association of Anatomists. 1997;247(2):289-98.
- research. 1913;27(4):425.
- Armour JA. Potential clinical relevance of the 'little brain'on the mammalian heart. Experimental physiology. 2008;93(2):165-76.
- Randall WC, Ardell JL. Selective parasympathectomy of automatic and conductile tissues of the canine heart. American Journal of Physiology-Heart and Circulatory Physiology. 1985;248(1):H61-H8.
- Randall DC, Brown DR, Li S-G, Olmstead ME, Kilgore JM, Sprinkle AG, et al. Ablation of posterior atrial ganglionated plexus potentiates sympathetic tachycardia to behavioral stress. American Journal of Physiology-Regulatory, Integrative and Comparative Physiology. 1998;275(3):R779-R87.
- Ardell J, Randall W, Cannon W, Schmacht D, Tasdemiroglu E. Differential sympathetic regulation of automatic, conductile, and contractile tissue in dog heart. American Journal of Physiology-Heart and Circulatory Physiology. 1988;255(5):H1050-H9.
- Dickerson LW, Rodak DJ, Fleming TJ, Gatti PJ, Massari VJ, McKenzie JC, et al. Parasympathetic neurons in the cranial medial ventricular fat pad on the dog heart selectively decrease ventricular contractility. Journal of the autonomic nervous system. 1998;70(1-2):129-41.
- Rajendran PS, Chui RW, Ajijola OA, Vaseghi M, Armour JA, Ardell JL, et al. Neural Control of Cardiac Function in Health and Disease. In: Dilsizian V, Narula J, editors. Atlas of Cardiac Innervation. Cham: Springer International Publishing; 2017. p. 13-35.